Abstract.
One of the most
important confirmation of the Big Bang theory was the discovery of the time broadening
of the light curve of the far supernovas (supernovas 1A, standard candles, at
the distances around Z=1). From straightforward consideration in the complete
absence of light scattering it can not be explained in any other way but by
Doppler-like effect which in this case called time dilation and seemingly
confirming the Big Bang. However, the hypothesis of tired light [1] also allows
the light being diffusion-like scattered on travel from supernova thus allowing
change in distance traveled and allowing corresponding time broadening of the
light curve (the fastest photons goes straight path, later arrived those which
due to multiple scattering – diffusion-like in perpendicular direction – first
traveled away from direct line, than returned back, possible many times and thus
got a big enough increase in distance to generate perceptible – few days-
delays at arrival). Problem with this mechanism is that gives smaller time delays compare to time dilation
directly observed [1]. The gravitational microlensing may be involved and due to additional change of distance,
Shapiro effect and brightening some parts of the image of supernova (3 separate
effects) will make the light curves
broader and thus explaining the time dilation observed.
Introduction.
Discovery of the
time broadening of the supernova type 1A (standard candle) light curves was
instantly interpreted as a proof of the Doppler time dilation (the processes
seems slower as a factor 1+z because the objects are moving away faster and
faster as they are further from Earth). Indeed, up to z~1 (the best Hubble may
do in supernova discoveries) the broadening of the light curve (time dilation)
follows this simple law (broadening~1+z) and it was considered as a final
confirmation of Big Bang. However, time dilation is also possible in tired
light theory (the photons experience diffusion-like process and some will
arrive few days later compare to the ones traveled the straight path). The real
difference between the time dilation as inferred from Big Bang Theory or from
diffusion-like tired light approach will be revealed at z~3 (and supernovas
with z~3 are already detected by James Webb Space Telescope, so the complete
light curve measurement may be on its way right now). This is because according
to Doppler-like time dilation the law 1+z will persist and at z~3 the time
dilation would be 4 times compare to z=0 (the light curve width for supernova
type 1A must be around 100 days instead of usual 24 days at z=0); while for
tired light the effect will be much smaller (light scattering quickly saturates
with z [2]) and width should be only ~40-50 days (more than 2 times narrower).
The difference between ~100 days and ~40 days is huge and for higher Z it will
be even larger – while the supernovas at z~12 in Big Bang cosmology must shine
bright around 260 days (more than half a year) the same supernovas for tired light idea will
shine for ~60 days (see how fast the scattering curve saturates in [2]).
Obviously if the time dilation will follow 1+z law up to those high z the Big Bang will be fully confirmed
and any tired light hypothesis completely eliminated. Interestingly this
observation is due in very close future – JWST is actually observing some
supernovas at z=3.8 already [3] and this is only the beginning. But for right
now it is necessary to explain how tired light may explain already observed
time dilation for supernovas with z~1.
Main part.
The
light curves of the supernovas are measured a lot, but for the further
supernovas some unexpected factors may contribute to the width and make the
curve broader what looks like time dilation. It may be influence of
gravitational lensing on a scale smaller
than generate the resolved multiple images (so called microlensing), with
corresponding Shapiro effect and strong influence of the wavelength of the
image taking detector since the light curve at one frequency is not the same as
light curve for different light color (and red shift at high z makes it even
more complex problem, it is necessary to recalculate light curve at different
wavelengths back to z=0). It may happened that the time dilation is a result of
multiple factors, which working together make the light curve broader than it
is.
At
first, the supernovas type 1A are not completely standard. They may be broadly
divided into brighter ones and dimmer ones [4]. Because of the range of white
dwarfs leading to explosion, some supernovas type 1A are brighter and some are
dimmer (but both are type 1A because of spectroscopic features). What is very
important for time dilation the brighter supernovas type1A are fading slower
(not very much but well resolved by the light curves). Since the brighter
supernovas are easier to spot at the high z (for Hubble supernovas at z=1 are already approaching the limit of
detection), the far supernovas have a selection bias toward the broader
light curve.
Another
important difference between the modern
model of the light propagation (no light scattering is allowed) and tired light
hypothesis is in the way the light propagating from supernova experiences
gravitational bending. The real size of supernova type 1A at the moment of the
maximum brightness (they usually discovered around this moment) is relatively small compare to typical
interstellar distances (distance Sun to Alpha Centauri is 3.8*10exp(16) meters).
The real size of supernova is only 2-3 light days at maximum brightness - something
like 3-4.6*10exp(13) meters [3] (just around 50 times larger than the giant red
stars with size up to 10exp(12) meters [5]). For this small size the beam
without light scattering may be bent by gravitational lensing but as a whole
(the right and left parts of the beam are experiencing the same path and the
same time delay both Shapiro effect and geometrical).
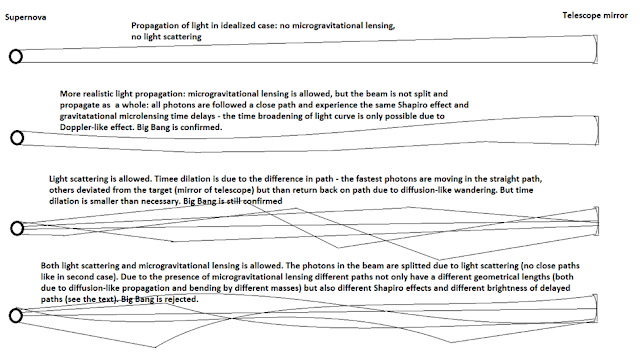
Due to the
presence of microlensing the real image of the supernova at Z=1 and beyond
would be consisting of many very small dots (provided the resolution of the
telescope is 100 times better than Hubble). This is because the smaller masses
(compare to strong gravitational lensing which generates visible Einstein
cross) will generate many Einstein crosses but they all blurred together due to
lack of the resolution. Interestingly the time dilation may be actually
observed even in the case of the absence of light scattering and absence of Big
Bang (no Doppler-like effect is necessary) – because the already observed
Einstein crosses are demonstrating huge difference in time of arrival of light
for 4 different images (up to 180 days) and possibly smaller, hardly resolved
Einstein crosses will give the time difference comparable to the observed time
broadening of the supernova light curves (if Einstein cross is not resolved,
all four images will give actually one but photons from different paths arrive
at different time, the observed light curve is actually of sum of 4 light
curves time shifted with respect to each other – it will inevitably be time
broadened).
In
[6] the excellent example of hardly resolved Einstein cross is shown with
lensing galaxy much weaker than the supernova images:
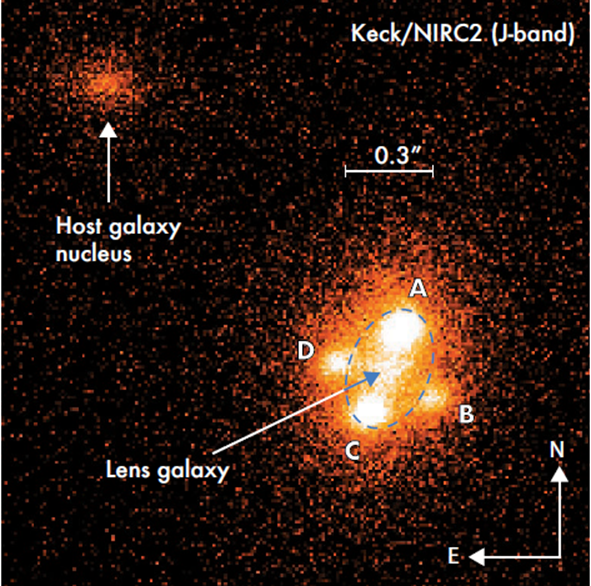
If the resolution of the
telescope would be just a little worse, this supernova would looks like one
image. But it would be actually consisting of 4 overlapped images with
different times of arrival and thus the observed light curve would be much
broader than each of the constituents. One of the explanations of the
supernovas huge visible angular sizes [7] (up to 6 times larger than
diffraction limit of the telescope at z=3 as observed by JWST) which preserves
the Big Bang is exactly this one – the real image is merely the
superposition of multiple Einstein crosses due to weak gravitational lensing
(unresolved because the resolution of
JWST is limited). In this case however the time broadening of light curves
(seemingly confirming Big Bang due to Doppler like effect) must be even more
pronounced – first because of Doppler effect (proportional to 1+Z) and second
due to overlapping of different images which have different paths and Shapiro
effects (proportional roughly to sqrt(Z)) – in total the time broadening of
light curves would be so big that the supernovas already at z=3-4 would shine
for many months. Such enormously large time dilation may be already dismissed –
even preliminary images of JWST (for z~3) made with time separation of months
are not showing such ultra-persistent supernovas.
The
last effect which may contribute the most (Shapiro effect is of course present
but usually considered as being around 10% of the time delay due to elongated
path) is the different brightness of the different constituents of unresolved
Einstein cross. Indeed, this is easy to see on the picture above – no Einstein
cross is ideal, usually one component is very bright and one is very dim. If
the Einstein cross is unresolved, this may contribute strongly to the observed
time broadening of the supernova making it very broad or very narrow (almost as
narrow as without any gravitational lensing effect). It also is different for
different wavelengths, making matter even more complicated. The great review on
this topic is [8] where the effects of microlensing for supernovas at z~1 were
estimated as leading to around 10-14 days difference in time broadening
(actually enough to explain the “time dilation” for supernovas even without any
Doppler-like effect).
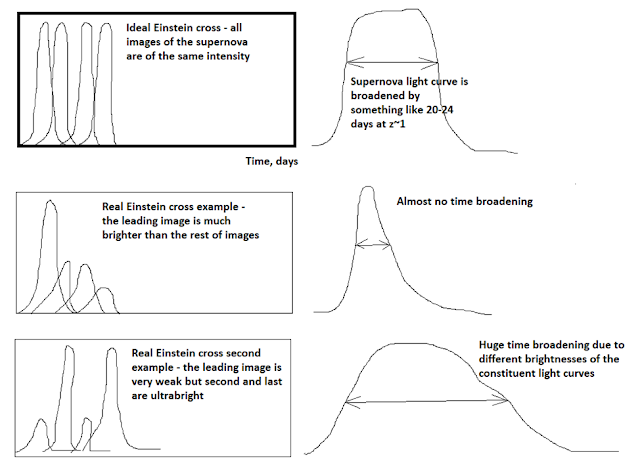
This effect is
mainly contributing to big scatter of the observed time dilations and should be
averaged on multiple observations of supernovae. Unfortunately to make such a
statistics even at z~1 thousands of supernovae are to be recorded with light
curves (unbearable task even for Hubble plus Earth based telescopes). And for
JWST so far only observations of the supernovae are made (and no reported light
curve is measured). Meanwhile this
effect by accident may generate extremely broad in time light curve for
supernova, seemingly confirming Big Bang, but in another accident may
completely reject Doppler-like effect – the scattering of data may be big.
Published data on light curves indeed confirmed the big scattering in observed
“time dilations” for supernovae at z~1, but whether it is due to such
brightness difference mechanism (of unresolved Einstein crosses) or due to
inevitable experimental errors is not clear at this time.
Conclusion.
While the
initial estimation of the time broadening of the light curve of supernova gave
a smaller than necessary value (4.4 days instead of 20 [1]) if other effects
are taken into consideration the tired light hypothesis may create big enough
value. As it is a typical case in many complex scientific issues, only direct
experiment may differentiate the Big Band and Tired Light in the question of
“time dilation”. Big Bang and Doppler-like effect must generate at least 1+z
time broadening (or may be even larger if microlensing and unresolved Einstein
crosses are taken into the consideration), while Tired Light must generate much
modest time broadening approximately like sqrt(z) (for more precise formula see
[9]). Already for z~3-4 (actual supernovae observed by JWST in 2023-2024) the
difference is huge and even with discussed brightness-generated errors should
be easy to differentiate. This experiment (direct measurement of light curves
for supernovae at z~3-4) may be considered as one of the simplest and possible
at the present time (beginning of the 21st century) ways to New
Physics (for more proposals see [10-11]).
References.
1.D.S.Tipikin “Tired light hypothesis possibly got
confirmation by direct observation of light scattering”
2311.0060v1.pdf (vixra.org)
https://vixra.org/pdf/2311.0060v1.pdf
2. Tipikin: Two galaxies (z=3.4 and z=14.32) are close together
on the JWST image - one is sharp, one is blurred. One more direct confirmation
of light scattering.
https://tipikin.blogspot.com/2024/08/two-galaxies-z34-and-z1432-are-close.html
3. Tipikin: Supernova's large angular size due to light
scattering for high z is clearly seen at multiple JWST images.
https://tipikin.blogspot.com/2024/08/supernovas-large-angular-size-due-to.html
4. Standard-Candle Supernovae are Still Standard, but Why? -
Berkeley Lab – Berkeley Lab News Center (lbl.gov)
5. Red giant stars: Facts, definition & the future of the
sun | Space
6. A.Goobar, J.Johansson, A. Sagues Carracedo “Strongly
lensed supernovae: lessons learned” // Philosophical Transactions A, 2024, 2406.13519,
https://arxiv.org/pdf/2406.13519
7.D.S.Tipikin “Comparison of angular sizes for supernovas
at z=0.151 and z=2.9 confirms the great resolution ofJWST and confirms the
presence of the light scattering. Tired light formula fits the angular size of standard
object like supernova surprisingly well on all distances.” // (PDF) Comparison of angular sizes for supernovas at z=0.151
and z=2.9 confirms the great resolution of JWST and confirms the presence of
the light scattering. Tired light formula fits the angular size of standard
object like supernova surprisingly well on all distances.
Or on Vixra: 2406.0162v1.pdf
( https://vixra.org/pdf/2406.0162v1.pdf
)
8. Daniel A. Goldstein, Peter E. Nugent, Daniel N. Kasen,
Thomas E. Collett “Precise Time Delays from Strongly Gravitationally Lensed
Type 1a Supernovae with Chromatically Microlensed Images” // 1708.00003
( https://arxiv.org/pdf/1708.00003
)
9.D.S.Tipikin
“Two galaxies (z=3.4 and z=14.32) are close together on
the JWST image - one is sharp, one is blurred. One more direct confirmation of
light scattering.” – published on Blogspot,
Tipikin: Two galaxies (z=3.4 and z=14.32) are close together
on the JWST image - one is sharp, one is blurred. One more direct confirmation
of light scattering.
https://tipikin.blogspot.com/2024/08/two-galaxies-z34-and-z1432-are-close.html
10.D.S.Tipikin “The quest for new physics. An
experimentalist approach” // 2011.0172v1.pdf
( https://vixra.org/pdf/2011.0172v1.pdf
)
11. D.S.Tipikin “Thee
quest for new physics. An experimentalist approach. Vol.2” // 2212.0058v1.pdf
( https://vixra.org/pdf/2212.0058v1.pdf
).